
High Gain Solar Plant in San Jose, California, the U.S.A.
By Bob Macdonald
Solar Market Segmentation
The market for large ground mount systems using silicon continues to evolve quickly, particularly in distributed generation applications where land is valuable. Thin film scales well into the tens of megawatts and works well in low light conditions, but efficiency and energy density are much lower than for high-efficiency silicon.
The ability to drive lower energy cost makes tracking important in sunny areas. For example, a large fraction of the PV system capacity installed in Spain in 2008 went into tracked systems and many of the large systems being installed in California, Southwestern U.S. and Southern Europe are now utilizing tracking technology. Other regions where tracking adds significant value include North Africa, the Middle East, Australia and parts of Asia.
Concentrating Solar Power (CSP) has attracted interest for very large, utility-scale deployments based on the prospect of low delivered energy cost and storage. If realized, this provides a measure of dispatch ability valuable to utilities. Obstacles to CSP deployment include large capital investment, very high water consumption, long design and deployment cycles, and transmission line issues.
Pre-engineered tracked PV systems combine the best aspects of tracked PV and tracked CSP and are a leading solution for sunny climates. HGS architecture, which is optimized for 100 kW to multi-megawatt applications, is one of the leading examples for this approach. The market segmentation for various technologies across system size and annual sunshine is graphically summarized in Figure 1.
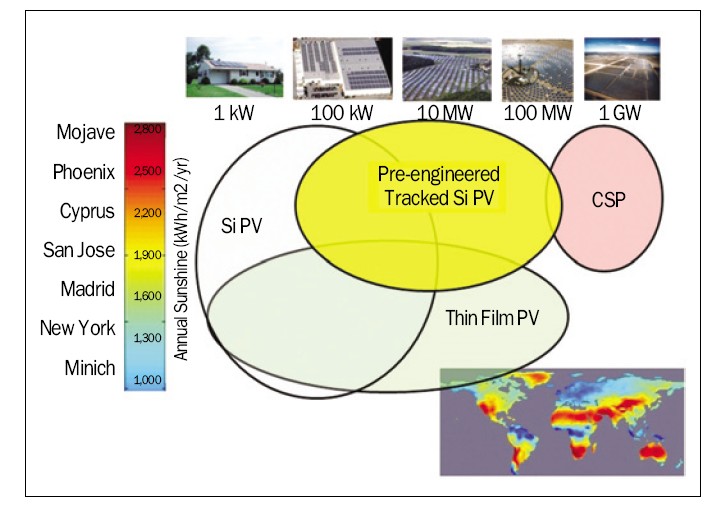
Figure 1. Solar market segmentation model (Source: Skyline Solar)
Opportunities for Better Economics
While most of the solar industry has focused on incremental efficiency gains for cells and larger cell manufacturing capacity, little attention has been paid to total system solutions which drive economic gains across the entire value chain. At a high level there are two paths to better economics as seen in Figure 2.
The first is to drive lower installed cost per peak watt ($/Wp). Cheaper and more efficient cells are a major part of this effort, but other opportunities include slashing costs, number of parts per Watt, low-cost panels, integrated racks and balance of system components (wiring, inverters), streamlining logistics and simplified installation. Thin film focuses on driving the overall cost per Watt peak down using low cost cells. It performs relatively well in less sunny climates like Germany, but its poor efficiency and requirement for large array area per Watt peak tends to drive the balance of system costs up and makes investment in tracking or other yield enhancements impractical in sunny climates.
The second path to better economics is through higher energy yield per peak watt (kWh/Wp). Tracking provides energy when it¡¯s needed most, in the high demand times of day, which provides more value for each kWh generated. Additional opportunities for higher yield include thermal cooling solutions, which lower cell temperature, better light capture, anti-soiling, improved string and shadow management and uptime.
Although many companies are starting to include these design elements in their product--cost notably, single-axis tracked high efficiency silicon systems--the traditional paradigm of component level optimization around flat panels has slowed the movement towards total system level optimization. For example, most panel vendors do not make trackers, and tracker vendors do not make panels. Panels are manufactured in the largest practical sizes, and trackers end up being generic (often over-engineered) designs that work for a range of commonly available panel sizes. Each set of vendors is constrained to working within its own sphere of influence, leaving the potential to rethink form factor, cooling and many other untapped opportunities for higher gain.

Figure 2. Opportunities for better economics (Source: Skyline Solar)
Technical Features
HGS takes the next step beyond traditional PV and CSP by combining the best concepts from each industry while fixing weaknesses of each, as seen in Figure 3. The architecture draws on trusted silicon cells but makes better use of them by reducing the amount of silicon required per watt. It also improves cooling to enable higher cell operating efficiency and tightly integrates the racking and tracking functions for simpler deployment and lower operating costs.
The system takes advantage of long reflective troughs and single axis tracking proven in the CSP industry over decades of operating experience. But unlike the heavy reflective structures (silver coated glass mirrors) used in early CSP, it uses sheets of low cost durable reflective metal encased in oxide layers to ensure high durability.
Because of their extensive water requirements, CSP systems have generally been conceived only for central power plants requiring large up-front design and capital investments. HGS is based on much smaller and more modular building blocks, which can be used in plants ranging from less than a hundred kilowatts to many megawatts with capital investment commensurate with size.

Figure 3. PV system evolution model (Source: Skyline Solar)
Reflective Rack for Efficient Output
The array contains a reflective rack, four rows of the panels running along the length of the array, and an integrated tracker in the system.
The reflective rack serves two functions; it provides structural support for the panels--similar to a traditional solar rack--and collects and reflects light from a large aperture onto the much smaller surface area of the panels. Initial systems have a concentration factor of roughly seven times.
Each reflective rack is constructed from four gently curved sheets of metal running along the top of the structure and a series of ribs and struts on the bottom. The top surfaces are covered with a thin highly reflective metal coating encased in oxide layers to ensure high durability. This technology was developed and tested over many years in the lighting industry. The overall structure forms a stiff, material-efficient space frame similar to structures used in the automotive and aircraft industries.
The reflective rack rests directly on posts without any additional framing. As a result, simple tracking actuators can efficiently turn long rows of modules at lower cost than conventional tracking systems. No gaps are needed between racks to accommodate supports, so arrays slot together forming a continuous collector surface which reduces twisting in high winds.
This design dramatically increases the effective output per gram of silicon and lowers the overall cost of the system. This is accomplished with little impact on the overall efficiency of the system and without introducing excess parts or fabrication steps that offset material savings.
The functional separation of light capture using large reflective racks, and conversion of light to electricity using small silicon PV panels allows HGS to benefit from innovation on two technology roadmaps at the same time--more efficient cells and better reflective materials.
The panels are manufactured with the same materials and processes as traditional solar panels however for the same energy output, they are roughly ten times smaller. The panels include a backing plate, silicon cells, encapsulant, glass cover plate and junction box. One unique feature of the panels is a metal heat sink, which allows passive convection cooling through open air channels.
This cooling solution allows the cells to run at similar or lower temperatures and higher efficiencies while traditional flat panels have no thermal solution and tend to lose efficiency during peak afternoon sun when heat trapped behind the panels drives cell temperatures up.
Integrated tracking enables the arrays to run at peak output through most of the daylight hours. This means they deliver more energy during peak afternoon demand when utilities charge their highest rates.

Figure 4. HGS design (Source: Skyline Solar)
Design Features
Pre-engineered Design
The rack assemblies are pre-engineered for simplified construction in the field. They have 50% fewer parts than a traditional tracked PV system because it is designed with pre-fabricated sub assemblies. In addition, the racks are designed to stack compactly in standard shipping containers and enabling efficient shipping from factory to field.
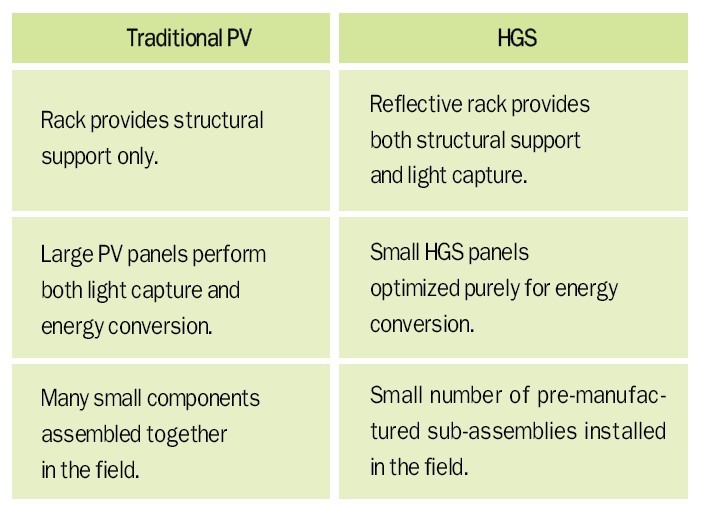
Figure 5. Traditional PV vs. HGS (Source: Skyline Solar)
Optimum Cell Design
The approach benefits from using a high efficiency PV cell. Standard monocrystalline silicon cells with a few modifications are used to optimize energy output for a moderate concentration system. A square PV cell made from one quarter of a 5-inch cell is used as well. Because the system concentrates light onto the cells producing higher current, components with less resistivity are preferred. For example, the metallization layer is designed to support high current densities. Because the array focuses light to within a few inches, the cell bus bars can be placed near the edge of the cell, minimizing any shadow effect. This design also provides more options for interconnecting the cells since the cells don¡¯t need to connect the cell from the back to avoid shading the cell.

Figure 6. Ratio of the measured vs. predicted power for a string of modules as a function of the roll angle—similar to solar angle (Source: Skyline Solar)
Integrated Predictive Model
The company has developed a performance model that uses empirical and analytical modeling to link environmental conditions to the efficiency of the components. The final model has been tested by comparing the measured power vs. the predicted power. Figure 6 illustrates the result of the ratio between the measured power and the predicted power for the system as a function of the roll angle over a period of two months.
The optical design for the system includes a trough with a reflective surface. The shape of the reflector has been optimized for large acceptance angle, light flux distribution for electrical and thermal characteristics, as well as optimum manufacturing tolerances. Figure 7 shows an example of an optimized the reflector delivering a very uniform flux distribution compared to a standard parabolic mirror. The uniform distribution of light across the PV cells avoids hot spots and produces higher energy output than a strictly parabolic design.
The system has been designed to reduce the variability of the light along the flux line. This results in an even distribution of light along the string of panels, minimizing current mismatch. Direct measurement methods are used to evaluate the shape of the reflector rack during manufacturing. Figure 8 shows the result of a laser-based system measuring a full array which is used for manufacturing screening, process development and failure analysis.

Figure 7. Flux distribution on panel for a standard parabolic trough compared to HGS reflective rack (Source: Skyline Solar)
Upgradable Design
The architecture is designed to be upgraded with the small PV panels. Since the PV portion of the system is dramatically smaller than a silicon or thin-film PV system, existing the systems in the field can be profitably upgraded to use new more efficient PV technology when it becomes available. This means that an investment in an infrastructure can be upgraded over time increasing the upside potential for generating more energy and revenue in the same space and extending the life of the solar power plant.
High Recyclability and Low Carbon Footprint
The environmental footprint created by the systems is smaller relative to traditional flat plate systems. Embodied energy and associated energy payback are key metrics used to judge the impact a technology has on its environment. Embodied energy is the total amount of energy required to build the system from raw materials through conversion. Energy payback is the period of time required for the system to generate that amount of energy by converting light to electricity. The system can be better than traditional PV on both counts as it is manufactured almost entirely out of recyclable metal. It uses substantially less silicon, which requires a high level of energy to manufacture, and less glass and encapsulant that are hard to recycle. At the end of its useful life, the majority of the metal content of the systems can be recycled and reused.

Figure 8. Experimental measurement of flux along an array using a laser beam method (Source: Skyline Solar)
Designed for Scale
The PV industry has recently focused on excess capacity and reduced materials cost, but there¡¯s a long-term opportunity to exploit system architectures that enable system, materials and production scalability. The system is part of the emerging class of pre-engineered, tracked PV systems whose manufacturing strategies rely only on currently existing silicon solar cell, module, reflector and aluminum parts supply chain as well as turnkey PV module production lines and metal fabrication industries that already exist at enormous scale. The reflector rack components are made with metal extrusions, stamps and die casts available in industrial parks around the world. The reflector sheets are produced from roll-to-roll sheets that are available in high volumes for lighting applications.
The manufacturing process follows an efficient path from rolled metal through stamping and robotic bonding and assembly. Manufacturing considerations that lower cost guide every facet of the design. This includes retaining integral fractions of standard cell and reflector widths; minimizing cuts, joints and machined features; and integrating racking and tracker support into the reflective rack.
Bob MacDonald is CEO at SkylineSolar (www.skyline-solar.com).
For more information, please send your e-mails to pved@infothe.com.
© www.interpv.net All rights reserved |